What is a safe CO level in our breathing air?
While articles in previous issues of Penyelam Siaga have addressed sources of carbon monoxide (CO) (Spring 2017) and prevention of breathing gas contamination (Spring 2014), perhaps less apparent to divers are the reasons why we have limits and the effects of exceeding those limits. This article will address the effects of CO poisoning and how the limits are derived. Discussions about the effects of gas need to consider the compressed gas, depth, gas uptake and dive profiles as well as the diver’s fitness level and general health.
Numbers are not absolute, need context to understand which are important and why, and might not apply equally to all divers. The effect of CO uptake at depth is not linear, nor do we know all the factors that may affect it, but we do know some things. Depth has a direct impact on partial pressure. Dalton’s law tells us that the partial pressure of all the constituents of the breathing gas in our cylinders will increase as we dive deeper, which means that the actual number of molecules per breath increases. It is a linear effect measured against absolute pressure in the same way as Boyle’s law addresses volume. A level of 10 parts per million (ppm) in a tank at the surface, for example, will translate to the same effect that 60 ppm has at 165 feet of seawater (fsw) or 6 atmospheres absolute (ATA) (10 ppm x 6 ATA = 60 ppm), which is referred to as the surface equivalent value (SEV).1
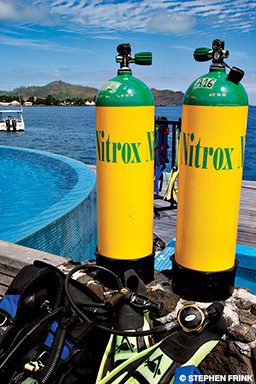
Producing nitrox by either gas separation (using a permeable membrane) or gas generator (using pressure swing absorption, or PSA) technologies further increases the number of CO molecules in the gas. Producing 40 percent nitrox using either of these techniques, for example, will increase the concentration of CO by a factor of up to three (meaning that we need three volumes of air to produce one volume of 40 percent nitrox).2 A measurement of 10 ppm in the air could thus result in up to 30 ppm in the cylinder. When we consider the SEV at a maximum diving depth of 80 fsw (3.4 ATA), the SEV would be 30 times 3.4, or 102 ppm.3 Complexities begin because the partial pressure of oxygen also increases, regardless of the breathing gas used. The deeper we dive, the greater the number of oxygen molecules. At 165 fsw we will have six times more oxygen molecules in each breath than at the surface while breathing the same gas mix.
The danger of CO in our breathing gas is the affinity of this toxin for our hemoglobin, which is the primary carrier of oxygen in the blood. CO binds at least 200 times more readily to hemoglobin and turns part of it into carboxyhemoglobin (COHb). The effect is that less hemoglobin is available to carry oxygen to the tissues. With the rise of COHb, tissues rapidly become starved of oxygen. The greater the number of CO molecules, the higher the percentage of hemoglobin turns into COHb (%COHb) and the greater the harm: We suffocate when the oxygen levels in our tissues drop too low.
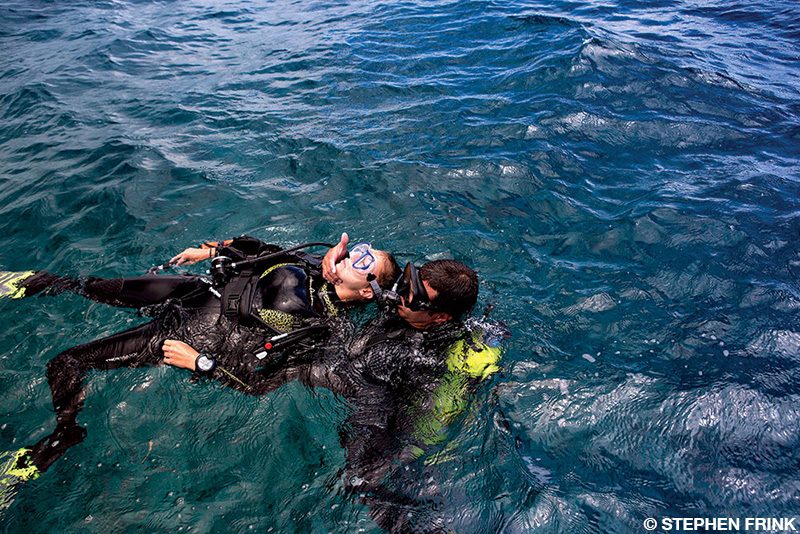
Other complex CO binding processes occur, causing further harm and long-term deficits in cellular respiration and energy production. A CO poisoning diagnosis considers the related symptoms, recent CO exposure and COHb levels. There is one mitigating factor: An increased partial pressure of oxygen dissolves into our blood (plasma), and despite the high percentage of COHb and decreased load of oxygen carried by remaining hemoglobin, this dissolved oxygen can keep our tissues supplied.
As we ascend, the partial pressure of oxygen and hence the dissolved oxygen will reduce. The amount of COHb, however, does not decrease at the same rate because it is a chemical bond and not a dissolved gas. It would typically take four to six hours to reduce the COHb level by half. Divers who breathe excessive amounts of CO may be asymptomatic at depth but rapidly develop symptoms when they ascend.
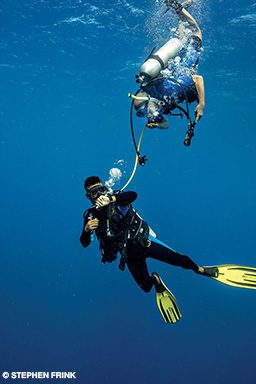
There is some debate and uncertainty over the relevance of the numbers. We would ideally be able to predict these effects so we can determine a safe level of CO in our cylinders. Time, the amount of CO, the diver’s breathing rate and general health condition are all part of the equation. While we do not have any significant research data on these effects in divers underwater, we do have the results of many occupational health and safety studies. Workers exposed to elevated levels of CO — which is possible in factories with power plants, furnaces, engine exhausts, certain chemicals and even in submarines — need to be able to safely complete their working day.
Table 1 contains some of the published data and safety levels found in a wide range of studies, regulatory documents and workplace standards.4 The differences in the actual amounts vary among sources, but the effects are similar.5

These COHb values are based on one-hour exposures with a respiratory minute volume (RMV) of 20 liters per minute (L/min). As exposure times lengthen to exceed eight hours, the COHb values will eventually plateau. The higher the RMV, the higher the %COHb for given concentrations of CO and time. RMV values measured in divers can range from 6 L/min to well above 35 L/min.
Table 2 shows the effect of depth on the potential toxicity of CO. Once again, the COHb values are based on one-hour exposures with an RMV of 20 L/min.

While both tables contain many numbers, the dramatic effect of depth on COHb and the rapid negative impact on health is clear.
We need to pay special attention to where the %COHb could rise above 30. The diver may lose consciousness at this level, which could cause drowning. Most of the accepted or required breathing gas limits for CO in your charged cylinder are either 5 ppm or 10 ppm.7,8 Both levels would be safe when considered for a dive time of around 60 minutes on air at the depths indicated in Table 2. Consider air with a CO concentration of 10 ppm in 40 percent nitrox. The resulting SEV of up to 102 ppm at 80 fsw (3.43 ATA) is in the danger zone, with a COHb content of approximately 14 percent (interpolated from the values in Table 2).
Divers with existing health issues, including impaired respiratory function, may be at a higher risk. For example, a one-pack-a-day smoker may live with a basal COHb level of 3 percent to 6 percent.9 Breathing a CO-contaminated nitrox mix could take COHb levels into the danger zone

A realistic standard that is safe, achievable and practical for most divers is 5 ppm. Many inexpensive, portable CO analyzers measure from zero to 25 ppm, with a 1 ppm resolution, making them suitable for detecting safe levels of CO in the dive environment.

Why 5 ppm when 10 ppm might also be safe? A maximum background level as high as 5 ppm is realistically possible. To achieve 10 ppm means there is likely a CO source nearby. While this level is perhaps safe, the compressor filling station needs to investigate the likely cause and assess the risk of drawing in CO of more than 10 ppm. Most operators do the required quarterly, biannual or annual air quality tests, depending on their legal requirements, but that doesn’t provide you much assurance about the level in your cylinder on the day you dive.
This article is not a thorough scientific analysis of the effects of elevated CO on divers, and DAN is not the appropriate organization to state limits. We also have not discussed what happens in technical diving, where the gas volume at depth contains a greater amount of CO. As the world’s leading dive safety organization, however, we advise that a maximum CO level of 5 ppm is relatively safe. While 10 ppm may be safe for diving, the value could feasibly change between filling sessions to levels that rapidly approach being dangerous. It makes little difference whether you are diving on nitrox or air.10 To ensure safety from the effects of CO, the ideal number and the one you should expect is zero.
Catatan
- The measurement parts per million (ppm) in this article is relative to volume.
- We assume an efficiency factor of 50 percent, which is typical for low-cost membrane separators.
- This assumes that the nitrox generator unit does not use a filtration system that includes an element to catalyze out the CO in the air. Catalytic converters are 99 percent effective at CO removal.
- Sources include the U.S. National Institute for Occupational Safety and Health (NIOSH), U.S. Environmental Protection Agency (EPA), U.K. Health and Safety Executive (HSE), New Zealand’s Health and Safety at Work Act (HSWA) and the World Health Organization (WHO).
- Despite the sources illustrating potential effects of elevated %COHb, a study in 2015 provided an even greater spread of the results of CO poisoning. There is thus no definitive correlation between %COHb in the blood and ill effects, as these effects vary between people. For this article and based on the regulatory sources cited earlier, this table serves as a guide until modified by future studies.
- COHb values are interpolated linearly between known values, but the correlation is only linear for the first few hours of exposure. Typical dive times allow for this. The values are rounded off to the nearest integer for values over 3.5 at the surface.
- EN 12021 (Europe), CZ275.2 (Canada), SANS 10019 (South Africa).
- CGA Grade E (USA), AS/NZ 2299.1 (Australia).
- A one-pack-per-day smoker may start the dive with a %COHb of 3 percent to 6 percent.
- While nitrox contains more oxygen, some people do not realize that producing nitrox using a membrane or molecular sieve separator does not remove the CO or the carbon dioxide. The effect is an increase in the amounts of both of these contaminants in the final gas produced — more oxygen but also more CO.
Daftar Pustaka
- Hampson NB. Myth busting in carbon monoxide poisoning. Am J Emerg Med 2016; 34(2):295-97. doi: 10.1016/j.ajem.2015.10.051.
- Rose JJ, et al. Carbon monoxide poisoning: pathogenesis, management and future directions of therapy. Am J Respir Crit Care Med 2017; 195(5):596-606. doi: 10.1164/rccm.201606-1275CI.
- Russel MAH. Blood carboxyhemoglobin changes during tobacco smoking. Postgrad Med J 1973; 49(576):684-87. doi: 10.1136/pgmj.49.576.684.
- Turner JA, McNicol MW, Sillett RW. Distribution of carboxyhaemoglobin concentrations in smokers and non-smokers. Thorax 1986; 41(1):25-27. doi: 10.1136/thx.41.1.25
© Penyelam Siaga — Q1 2020