“A number of factors contribute to your individual susceptibility to DCS and can even alter your susceptibility from day to day.”
The most significant risk factor is your exposure profile — that is, the time, depth and ascent rate of your dives. Some degree of exposure intensity is required to initiate a decompression insult, regardless of the presence of other predisposing factors.
There are a host of factors, however, that can play a role in your outcome if you experience an exposure sufficient to make DCS a possibility. Several common risk factors are outlined in this chapter.
In this chapter, you’ll learn about:
- Workload
- Thermal Stress
- Optimal Practices
- Postdive Air Travel
- Medical and Physical Fitness
- State of Hydration
- Breathing Gas Mixture
- Carbon Dioxide Level
- Patent Foramen Ovale
- Additional Factors
Workload
During the Dive
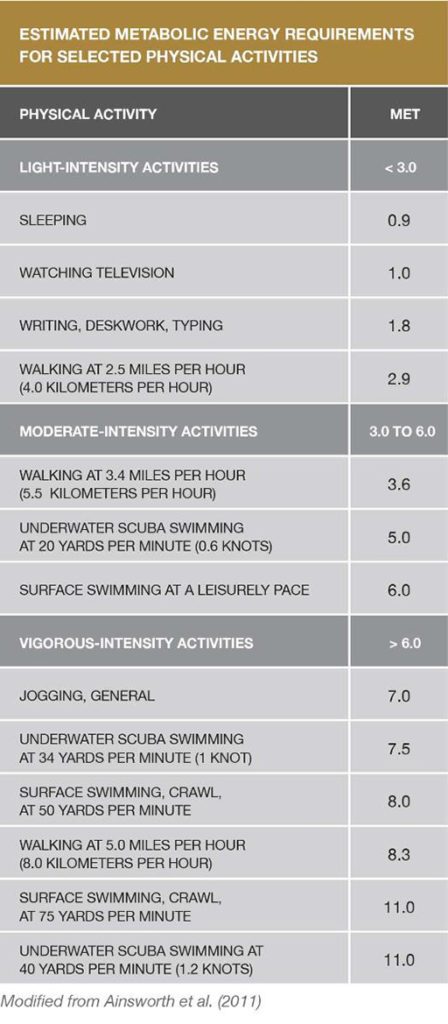
The timing and intensity of exercise during a dive can substantially affect your risk of DCS. A high workload during the descent and bottom phase of a dive will increase your inert gas uptake, effectively increasing the subsequent decompression stress. And exertion near the end of or immediately after a dive, particularly if it involves high joint forces, can stimulate bubble formation and increase the likelihood of bubbles passing through the lungs without being filtered out of the circulation.
You should keep your exercise intensity as low as possible during the bottom phase of a dive. Mild exercise — on the order of no more than two to three times resting effort, and with very low joint forces — is appropriate during the upper ascent and stop phases of a dive. However, any exercise, particularly exercise involving high joint forces, should be avoided as long as possible after a dive. If you are unable to avoid postdive exercise, you should keep your dive profiles very conservative to minimize your overall risk.
Thermal Stress
A diver’s thermal status has long been known to influence decompression risk. The impact is best appreciated by considering the two fundamental phases of every dive: the descent and bottom phase, when gas uptake occurs, and the ascent and stop phase, when gas elimination occurs.
Two Phases
During the descent and bottom phase of a dive, a relatively warm state results in increased inert gas uptake; this is equivalent to conducting a deeper and/or longer dive. On the other hand, if you can maintain a cool or thermoneutral state during your descent and bottom phase, you will effectively reduce your inert gas uptake. This beneficial effect will be further magnified if you exert yourself as little as possible during this phase.
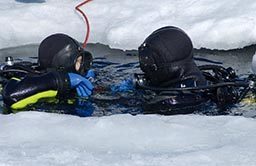
During the ascent and stop phase of your dive, a relatively warm state will promote inert gas elimination, thus reducing overall decompression stress. On the other hand, a cool or cold state during this phase will reduce inert gas elimination, effectively prolonging and possibly increasing decompression stress.
The decompression hazard associated with hot water suits — which effectively establish a warm condition in both phases of a dive — was established in a study of North Sea divers conducted 30 years ago (Shields and Lee 1986). The impact of thermal status on decompression stress was even more elegantly demonstrated in a recent study conducted by the U.S. Navy (Gerth et al. 2007). The controlled conditions of a research study cannot be directly correlated with everyday diving practices, but the key message from these studies is the importance of thoughtful thermal status. Keeping neutral on your way down — certainly avoiding unnecessary overheating — and warm on your way up (approaching a cool-warm pattern) will reduce the risk of DCS in comparison to being warmer on your way down and cool on your way up (a warm-cool pattern).
Optimal Practices
The difficulty comes in reconciling optimal practices for decompression safety with divers’ desires and normal practices. It is understandable for divers to want to warm themselves before the start of a dive, in anticipation of getting colder as the dive proceeds. Historically, divers did this by pouring warm water into their wetsuits or gloves before a dive. Then some divers began to place chemical hot packs in their suits. Modern divers have even more choices available to them, due to today’s array of active heating garments suitable for use with either wetsuits or drysuits. The problem, though, remains the same: warming the body’s peripheral tissues enhances circulation and increases the delivery of inert gases, particularly if the heating is applied early in a dive, when inert gas uptake is typically at its highest level. Furthermore, both warm water and chemical hot packs lose their effectiveness over time, potentially creating the warm-cool pattern shown to generate the greatest risk of DCS. Even active heating garments — which are able to keep the diver warm throughout a dive — involve a somewhat elevated risk. As shown with hot water suits, a warm-warm pattern, while associated with less DCS than a warm-cool pattern, remains more hazardous than a cool-warm pattern. Practically, divers should maintain adequate thermal protection to ensure clear thinking and physical capability. Excessive warming during dives should be avoided.
Divers must also keep in mind that postdive warming can also influence decompression risk. Indulging in rapid postdive warming, such as by taking a hot shower or getting into a hot tub, decreases the solubility of inert gas in tissues. This will promote the formation of bubbles in local tissues, often before perfusion increases sufficiently to remove the gas. Skin symptoms, fortunately often mild and transient — not cutis marmorata — can develop with rapid warming of the skin postdive. The challenge is to get divers to prioritize safe decompression over pure comfort. If an active heating system is to be used, this means leaving it off or on its lowest setting during your descent and bottom phase, and then turning it up a modest amount during your ascent and stop phase. It also means delaying the postdive pleasure of jumping into a hot shower or hot tub. If delayed gratification is not your style, then you should use more conservative dive profiles to reduce your overall risk.
Postdive Air Travel

Modern air travel has made distant dive locations easily accessible. Flying to a destination near sea level before diving engenders virtually no risk (outside the possibility of mild dehydration or impairment due to long periods of relative immobility). Since flights end with compression, the tissues of plane passengers will be undersaturated upon landing and subsequently accumulate inert gases to re-establish equilibrium with the ambient pressure.
Flying after diving, however, increases decompression stress, since the pressure in an aircraft cabin is lower than that of ground-level atmospheric pressure. Commercial aircraft must have the capability of maintaining cabin pressure at an equivalent of 8,000 feet (2,438 meters), approximately 0.76 ATA. This does not mean that cabin pressure is always maintained at higher pressures. A recent study found that 10 percent of the commercial flights tested had cabin pressures exceeding 8,000 ft (Hampson et al. 2013). Now imagine that you have just completed a dive to 66 feet (20 meters), where you experienced an underwater pressure of 3.0 ATA. Your return to the surface, and the 1.0 ATA pressure of sea level, has already subjected your body to a threefold reduction in pressure (3.0:1.0). If you then get on a plane that has a cabin altitude of 8,000 feet, you would be subjecting yourself to a fourfold reduction (3.0:0.76) and thus to even greater decompression stress. Furthermore, should your plane suffer an unlikely but not impossible cabin depressurization, you would be subjected to a much greater decompression stress.
DAN and the Undersea and Hyperbaric Medical Society (UHMS) held a workshop in 2002 to review the available data regarding the decompression stress of flying after diving and develop consensus guidelines (Sheffield and Vann 2004). There were two important stipulations regarding the guidelines: first, adhering to them will reduce your risk but offers no guarantee that you will avoid DCS, and second, observing even longer surface intervals than the recommended minimums will reduce your DCS risk further still. Keeping in mind these caveats, these are the guidelines:
- After a single no-decompression dive, a minimum preflight surface interval of 12 hours is suggested.
- After multiple dives per day or multiple days of diving, a minimum preflight surface interval of 18 hours is suggested.
- After dives requiring decompression stops, there was little evidence on which to base a recommendation, but a preflight surface interval substantially longer than 18 hours is considered to be prudent.
There are two further factors of note regarding the DAN-UHMS flying after diving guidelines:
- They apply to flights at altitudes of between 2,000 and 8,000 feet (610 and 2,438 meters). The effect of a flight at an altitude below 2,000 feet was considered mild enough not to warrant special consideration — giving divers the flexibility to engage in modest postdive air travel, such as a short, low-altitude, inter-island flight.
- They apply only to divers who have experienced no DCS symptoms. It is essential that a diver who is experiencing any symptoms consistent with DCS seek treatment prior to flying.
It is important to remember that any postdive ascent to a higher altitude — even using ground transportation — increases your decompression stress. Taking a cautious approach in such a case, by keeping your final dive profiles more conservative and/or delaying your travel to the higher altitude, is always advisable. The U.S. Navy has generated detailed tables and procedures that allow computation of exposure limits to a greater range of altitudes and with more time flexibility than the DAN-UHMS guidelines (USN 2008). It is important to appreciate, though, that these are simply mathematical constructs based on the same data used in developing the DAN-UHMS guidelines. Furthermore, they require the computation of repetitive groups for planning, something that is done with dive tables but not dive computers. Despite these limitations, they can be useful, particularly for a regular pattern of altitude diving.
Medical and Physical Fitness

Poor medical and physical fitness can compromise your safety in general and may increase your risk of DCS. Definitive data are limited, but there is no question that it is prudent to maintain a high level of physical fitness and to dive progressively more conservatively as your fitness level declines. Safe diving is possible throughout much of a normal life span, but it is important for all divers to seek regular, objective evaluation of their capabilities and to adapt their diving practices accordingly. But even for divers who have transitioned from independent to more dependent forms of diving, in which they increasingly rely on the support of others, there will ultimately be a point at which they should hang up their fins.
Physical Activity Recommendations
Adults need two types of regular activity to maintain or improve their health—aerobics and strength training. The Centers for Disease Control and Prevention’s 2008 Physical Activity Guidelines for Americans recommends at least two and a half hours a week of moderate-intensity aerobic exercise to achieve health benefits, and five hours a week to achieve additional fitness benefits. And just as important as engaging in aerobic exercise is doing muscle-strengthening activities at least two days a week.
While good health and physical fitness will not solve all problems, the foundation is an important one. An adequate physical reserve can allow a quick response to keep a small problem from becoming a serious one. Relevant scenarios can be easily imagined for almost any dive.
Regular aerobic exercise has many positive benefits. Cardiac reserve is the difference between the rate at which the heart pumps blood at rest and its maximum capacity. An increase in this reserve may make it easier to meet the physical demands of diving activity and stress. Blood values of cholesterol can improve, reducing susceptibility to heart disease. Insulin sensitivity can improve, reducing the risk of developing diabetes. While the data specific to diving are much more preliminary, there is also some evidence that higher levels of aerobic fitness may contribute to a reduced decompression stress.
Most individuals are aware that being fit can improve quality of life. A major problem, however, is that time takes a toll on us. The ease with which we maintain our fitness level in our 20s can be very different from the reality as decades pass. Aerobic fitness typically declines on an average of one percent per year after age 30. The important point is that while some decline may be unavoidable due to a gradual loss of muscle mass and a reduction in the metabolic activity of aging muscle, the rate can be slowed and the reserve range broadened by adopting healthy lifestyles as early as possible.
The physical fitness needed for diving will vary with the demands of the environment, the equipment, and the nature of the dive. The best strategy is to incorporate regular physical activity into your life to improve or preserve your capabilities, and to prolong your diving life. Do not count on diving to keep you physically fit. If done properly, it should be your relaxing time in the water. To maintain or build aerobic capacity and strength, swim, cycle, run, or do whatever other physical activities you can enjoy. The more fit you are, the longer you get to play.
Detailed physical activity recommendations can be found at cdc.gov/physicalactivity/everyone/guidelines.
State of Hydration

Dehydration gets a substantial amount of attention in the lay diving community as a risk factor for DCS, but probably more than is warranted. Sound hydration is important for good health, both for general and for diving health, but for your dive profile, thermal stress and exertion level are far more important risk factors for DCS. The undue focus on dehydration is probably a result of two issues. The first is that substantial fluid shifts can result from DCS, often creating a need for substantial fluid therapy and creating an impression that this was a cause, rather than a consequence, of the disease. The second issue is human nature — the understandable desire to assign blame for a condition that is capricious. DCS is fickle. A diver may adhere to a similar dive profile many times without incident but then develop DCS while following the very same profile. It is comforting to try and identify a single causal agent, even if this is more wishful than factual. It is important for divers to realize that a multitude of factors can subtly affect the risk on any one dive and that there is a probabilistic nature to the disease. Focusing on a range of strategies to reduce risk is more effective than trying to put all the blame on one.
Breathing Gas Mixture
The particular breathing gas mixture you use, and how you use it, can play a role in the development of DCS. A mixture known as enriched air nitrox, or simply nitrox, is increasingly popular for recreational diving. The percentage of oxygen in the mix is increased, reducing the nitrogen fraction. This means that there is less nitrogen uptake at a given depth. The decompression effect of nitrox, compared to that of air, can be calculated by computing what is known as equivalent air depth (EAD). The risk of DCS when diving with nitrox to the EAD table limits is not appreciably different than diving with air to the air table limits. It is possible to achieve a decompression safety buffer by using nitrox with air table limits, since this will reduce your inert gas uptake compared to using air.

The critical caveat with nitrox is that its higher oxygen content means that a diver breathing nitrox is at risk of developing oxygen toxicity at a shallower depth than a diver breathing air. The recommended maximum partial pressure of oxygen — partial pressure being the portion of the total gas pressure represented by a single gas — is 1.4 ATA for recreational diving. When diving with air (21 percent oxygen), this level is reached at a seawater depth of 187 feet (57 meters) — beyond the usual recreational diving limit (187 feet of seawater = 6.6 ATA * 0.21 ATA oxygen in air = 1.4 ATA). When diving with a 32 percent nitrox mixture, this level is reached at a seawater depth of 111 feet (34 meters), and with 36-percent nitrox at just 95 feet (29 meters) — depths commonly reached by recreational divers.
Carbon Dioxide Level

Elevated levels of carbon dioxide can increase the risk of DCS and lower the threshold for oxygen toxicity. Carbon dioxide is a potent vasodilator, meaning it causes the blood vessels to widen, increasing blood flow and the delivery of gases to tissues. Factors that can raise divers’ carbon dioxide levels include the increased dead space of breathing equipment (gas volume that must be moved but does not take part in gas exchange), the additional work of breathing dense gas underwater, and exercise. Using a well-designed and well-maintained breathing system, minimizing physical effort and remaining relaxed while underwater can minimize carbon dioxide increase.
Patent Foramen Ovale

Patent foramen ovale (PFO), literally, open ovale window, is a persistent opening between the left and right atria of the heart. In fetal circulation, a major opening between the atria allows blood to largely bypass the lungs that are not yet being used for gas exchange. A flap normally closes over the opening after birth and is sealed by tissue. In approximately 25 percent of the population, a partial opening remains, the PFO. The opening can range in size from functionally irrelevant to physiologically significant, the latter allowing a substantial portion of blood to be shunting from the right heart to the left heart, bypassing gas exchange and filtration in the lungs. PFOs typically produce no symptoms and individuals are unaware of their status unless they are incidentally discovered through medical tests. However, the presence of a large PFO may increase the risk of DCS in divers who develop significant bubble loads. The correlation between PFO and DCS risk is not a clear one, since the frequency of PFO in the population is fairly high while DCS is relatively rare. The safest strategy — even if you have not been diagnosed with a PFO, but most certainly if you have — is to dive in a manner calculated to keep your bubble load low; this effectively eliminates any concern that bubbles might pass through a PFO and bypass the lungs, where they would normally be filtered out.
The most commonly held consensus is that screening all divers for PFO is probably not warranted. And even in divers who have been diagnosed with a PFO, deciding whether it warrants surgical closure is a choice that each individual should consider carefully with a well-informed medical team.
Additional Factors

A host of other factors may also contribute to any given individual’s risk of DCS. Some probably play minor roles, and some potentially play important roles that have not yet been fully defined. Nutritional status, for example, plays a major role in one’s general health and often in one’s physical fitness, too. While research on the subject of nutrition and diving is limited, it is possible that it also affects decompression safety. For example, one study assessed the relationship between cholesterol levels and decompression-induced bubbles. Doppler ultrasound was used to classify the 30 subjects as either “bubble-prone” or “bubble-resistant.” Among the study’s findings was that, on average, bubble-prone subjects had higher total blood cholesterol levels than the bubble-resistant subjects (Webb et al. 1988). Additional research into this and many other areas is needed.
Sex
There is little evidence in the diving medicine literature that sex plays a role in the development of DCS. Even if women do have a slightly elevated risk, as is suggested in the aviation medicine literature, it is possible that making safer choices with regard to your diving practices can compensate for any slightly elevated physiological susceptibility.
Age
Advancing age is sometimes suggested to increase DCS risk, but it may simply reflect typical patterns of compromised physical and medical fitness.