“Divers are surprised when symptoms of DCS develop after dives that appeared safe according to their dive computers. Remember, models reflect an average diver, not you.”
In recent years, dive computers have supplanted dive tables as the primary means of regulating dive profiles. Dive computers offer an advantage in that they enable the diver to dynamically establish different compartments as the controlling compartment, as conditions change during a dive. In reality, the compartments in a dive computer’s modeling software do not have to represent any particular tissue, as long as the guidance provided by the model results in an acceptable outcome — specifically, very little DCS.
In this chapter, you’ll learn:
Important Cautions
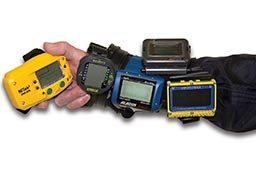
While the guidance provided by decompression models can be very useful, it is important for divers to keep in mind that dive schedules — whether they are presented in printed tables or on the screen of a dive computer — are limited in what they measure and in the assumptions upon which the model was constructed. Tissue compartment parameters can be adjusted, or new compartments can be added to an algorithm, if experience shows deficiencies in a given model — but in real time, the calculations are limited by the variables that are being processed. Algorithms can estimate limits based on time and pressure (depth) profiles for a given breathing gas, but they are not able to compute the impact of myriad real-time factors, including thermal status, exercise intensity, joint forces and a host of individual predispositions that are currently not well understood, let alone quantifiable in their impact on decompression stress.
Divers are often surprised when symptoms of DCS develop after dives that were conducted within the limits of their dive computers. It is important to remember, though, that while mathematical models predict outcomes, they do not guarantee them. The fact that a dive was conducted within the limits suggested by a dive computer (or a dive table) does not make a DCS hit “undeserved.” The mathematical algorithms provide guidance that must be evaluated and tempered by a thoughtful diver.
Many divers are also unaware of the fact that dive computers make use of many different mathematical models, or versions of different models; there is no universal standard. A single manufacturer may even use more than one model, possibly in a single type of computer. This makes it extremely difficult to assess the nuances of every system.
Basic Guidelines
There are some basic guidelines that can help to ensure the safe and effective use of a dive computer. The following considerations are intended to offer a somewhat light-hearted insight into what your dive computer can — and cannot — do.
It is helpful to think of your dive computer in these ways:
- As a business competitor: Master it by learning its strengths and weaknesses.
- As a date: It must be turned on for the relationship to work.
- As a buddy: It should descend and ascend whenever, but only when, you do.
- As a personal assistant: It reminds you of rules and schedules you might otherwise forget.
- As an actor: It recites the lines without having to understand their implications.
- As a politician: Do not believe everything it tells you.
- As a hotel concierge: It will help you do what you want — but at a price.
- As a stranger: It knows virtually nothing about your personal reality.
- As a mate: Is it compatible with your friends?
- As a news reporter: It will air your dirty laundry.
- As a tool: Use it appropriately.
Specific Tips and Tricks
Push the Right Buttons
You should know not only which buttons to push to make your computer work, but also which mathematical model or model derivation it employs in making its decompression computations. There is a surprising range in models, from conservative to liberal, and these differences may not be evident at first glance. For example, a computer may establish conservative limits for an initial dive but liberal limits for repetitive diving. It is best to learn enough about the various available models and derivations before you select a dive computer, so you are sure to choose one that is compatible with your own level of risk tolerance. Choosing one purely based on familiarity may not be the best strategy. Even if you have had good outcomes on previous dives with a computer, it does not guarantee that it will be the best one for your future diving. Accumulating knowledge takes commitment, but informed planning for decompression safety should be a top concern.
Tune In and Turn On
Failing to turn on your dive computer (or to take it with you on a dive) may sound like a joke, but it does happen and can create real problems. No computer can factor in the exposure profile of a previous dive if it was not there. And any decompression model is invalid unless you start using it when you are “clean” — fully off-gassed from any previous dives. If you forget to take your computer with you on a dive early in a repetitive series, you are then restricted to using tables for the duration of that series (assuming that you are able to manually compute the exposure of the unmonitored dive). And do not even think about hanging your computer on a downline during a surface interval in an effort to compensate for having forgotten it on an earlier dive; there may be stories about that happening, but it is not a responsible practice.
Use It Appropriately
The only person who does not have to worry about taking a dive computer on every dive is the one who uses it solely as a datalogger — that is, only to record time and depth information instead of to calculate decompression profiles. Remember, however, that using your computer simply to log your time and depth data means that you must still plan all your dives using dive tables and must recompute your repetitive group status afterward, as appropriate. You cannot move in and out of relying on your computer’s decompression computations unless it has recorded all of your exposure profiles.
Remember Its Limitations
Dive computers are wonderful at carrying out programmed mathematical computations, but they are blind to the many insights you may have before, during and between your dives. For example, your dive computer knows nothing about your personal health status, your level of physical fitness or your individual susceptibility to decompression stress. It also knows nothing about your thermal stress or physical efforts during or between dives. The fact that many dive computers display water temperature might suggest that thermal stress is factored into the device’s algorithms. A water temperature reading, however, provides no useful information regarding thermal stress, since the diver carrying the device could be wearing anything from a bathing suit to a wetsuit without a hood to a cold-water drysuit with a hood, gloves and cold-water undergarments. More important, it is not yet possible to directly compute the impact of differences in thermal status during different parts of a dive, even if the computer was able to measure the diver’s core temperature and skin temperature in key spots.
We do know that being warm (rather than cool or cold) during the compression and bottom phase of a dive promotes inert gas uptake (not optimal), and that being warm during the decompression phase promotes elimination (optimal). While impractical for the comfort-loving diver, decompression safety is optimized by being neutral or cool during the inert gas uptake phase of descent and bottom time and warm during the inert gas elimination phase of ascent. While the concept of thermal changes on decompression stress is clear, we are still years away from being able to quantify the real-world effects of these factors for dive-planning purposes. Similarly, while some computers are able to track gas consumption, we have much to learn before this information can be meaningfully incorporated into decompression models. Variations in air consumption can reflect differences in the depth of a dive or in the diver’s experience, level of anxiety or degree of physical exertion. The bottom line is that interpreting the precise physiological impact of the interactions among these diverse factors is exceedingly difficult, requiring thoughtful practice by divers.
Heed Your Computer’s Readings
Divers need to pay attention to their dive computers if the information provided is to be of any use. Be aware that confirmation bias can promote risky behavior. “Getting away with” a risky exposure once, twice or even many times may eventually catch up with you. It may not truly be safe for you or for a partner who might have a higher degree of susceptibility to decompression stress. Those who wish to worry less about their exposure will have greater peace of mind if they choose a computer that employs an extremely conservative decompression model. It is also important to pay attention to your dive computer. If you are diving with a group, do not forget that there can be considerable variability in the guidance provided by different computers or computers with different user-selected settings. That means there is considerable benefit in diving with others who use a computer with a similar decompression model and settings, because if modest discrepancies arise, following the most conservative directive will likely not be terribly burdensome for the group. But if members of a group are using dive computers with substantially different models, and each diver wishes to follow his or her own device, it can lead to a breakdown in the buddy system.
Do Not Rely Blindly On Your Computer
Although heeding your computer is important, do not take its advice unthinkingly. The same profile can sometimes be conducted without problem again and again, right up to the dive where it does not prove safe. Divers often try to blame a specific factor, such as dehydration, for the development of symptoms following one dive but not another. This approach is not productive. The range of variables in play during a dive are rarely identical, and there is a probabilistic element to decompression risk — that is, chance can play a role in the manifestation of DCS.
The best approach is to avoid the extremes of either fatalistic resignation or smug focus on a single supposed magic bullet. There are many, many small steps you can take to make any dive safer. The most important one is to stay within a reasonably conservative time-depth profile and to add safety stops to every dive. Other important steps are to minimize your exercise intensity and avoid overheating during the gas-uptake phase of your dive, to choose the right breathing gas, to practice enough that you are able to perfectly control your buoyancy, to remain well-rested and well-hydrated, choose more conservative user-adjustable settings on the computer, and to dive with a partner who has similar goals and follows similar practices. Adding small safety margins to each step can help to provide a comfortable security cushion. Dive computers are powerful tools, but sound knowledge of diving physiology, good physical conditioning and adherence to thoughtful practices offer the best protection for divers.
Keep It With You
If you do develop DCS symptoms, you should keep your computer with you when you go for medical evaluation. Some facilities may have the ability to download or review your profile to aid in the evaluation of your case. The medical staff will surely appreciate seeing confirmation of your description of the events that precipitated your symptoms.